Welcome to MiSciWriters!
We are a graduate student organization at the University of Michigan whose goal is to train fellow graduate students & post-doctoral fellows in science writing and editing.
Science communication has always been important, but more recently it is stepping into the spotlight with more academics either leaving the bench or being involved with their communities more while remaining a researcher. We can help you regardless! By practicing your writing and editing skills, you’ll gain experience that’ll help write grants, give public talks, or write journalism articles all while meeting and networking with like-minded and interested sci-commers in the community.
Check out the Blog page to read our articles (where a few & increasing number have been translated into Spanish).
To learn more about science writing, we have a bunch of resources and outline our editorial strategy here. We welcome any level of writer, so we hope that you reach out!
The inaugural edition of our STEAM magazine, equilibriUM, is now available! Click here to read!
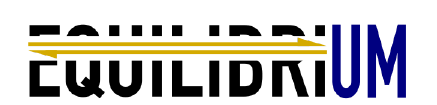
We are generously sponsored by the Rackham Interdisciplinary Workshop grant. Our published magazine has various departmental sponsors, which can be found here.
Thanks to Callie Corsa for making our logo!